Analysis of Nicotine and Impurities in Electronic Cigarette Solutions and Vapor
Abstract
Electronic cigarettes (e-cigarettes) are growing in popularity exponentially. Despite their ever-growing acceptance, relatively little work has been done to characterize their vapor. To date, the majority of e-cigarette research has focused on characterizing the solutions, which are ultimately vaporized for the end user to inhale. The current study focused on developing a complete analytical package for the quick and simple analysis of electronic cigarette solutions and vapor to determine nicotine content and impurity profiles. Rapid (<5 min) gas chromatography–flame ionization detector (GC-FID) methods (using both helium and hydrogen carrier gas) were developed for the determination of nicotine content in e-cigarette solutions. In addition, a straightforward GC mass spectrometry (GC-MS) method was developed for the determination of impurities in e-cigarette liquids. Lastly, a simple sampling device was developed to draw e-cigarette vapor into a thermal desorption (TD) tube, which was then thermally extracted and analyzed via the same GC-MS method. This novel approach was able to provide detectable levels of volatile organic compounds (VOCs) and semivolatile organic compounds (SVOCs), which were not detected in the liquids, from a single 40 mL puff. All three of the methods may be done with one GC, two detectors, and one analytical column (Rtx-VMS), thereby reducing required resources and affording easy comparison of results.
Introduction
Electronic cigarettes (e-cigarettes) do not burn tobacco, rather they produce an aerosol (without flame or smoke) from a battery-powered, metal heating element and liquid-containing cartridge [1]. The liquid typically consists of humectants (propylene glycol [1,2-propanediol] and/or glycerin), flavorants, and nicotine [2]. When an e-cigarette’s power source is activated, the heating element vaporizes the liquid to form a mist, which the end user then may inhale (often referred to as “vape”) [3]. The smoke-like vapor imitates tobacco smoke visually and replicates the burning sensation in the throat and lungs (often referred to as “throat hit”). These similarities to tobacco smoke, combined with the same hand-to-mouth behaviors, have contributed to the rapid adaptation of electronic cigarettes [4-6]. Despite their increasing use on a global scale [3], relatively little is known about the e-cigarette chemical components. The majority of studies have focused primarily on the nicotine content and impurities (e.g., nitrosamines) of e-cigarette liquid (e-juice) [7]. More important, relatively little is known about the chemical composition of the vapor, which is ultimately what end users are exposed to [7, 8].
Only a few researchers (e.g., Goniewicz et al. [7], Kosmider [9], and Schober et al. [8]) have attempted to characterize e-cigarette vapor by analyzing it for the presence of volatile organic compounds (VOCs), nitrosamines, heavy metals, and polycyclic aromatic hydrocarbons (PAHs); however, their study designs have been relatively complex and/or required the use of a specialized smoking machine and/or an array of specialized analytical instruments. Such requirements are often not practical for routine contract laboratory testing. The current study evaluates the nicotine content and impurities of several commercially available e-cigarettes and their respective solutions via simple and rapid GC-FID and GC-MS methods. In addition, the primary e-cigarette emissions were analyzed for VOCs and semivolatile organic compounds (SVOCs) via a simple and novel technique that pairs thermal desorption (TD) with GC-MS. Results, analytical techniques, obstacles, and solutions are discussed.
Experimental
Electronic Cigarettes and Liquids
Four commercially available electronic cigarettes (Table I) were chosen from the “Best E-Cigarettes of 2014,” which is a top 10 list of e-cigarettes as viewed by “experts and users” [10]. It is important to note that these four chosen e-cigarettes also routinely appeared on other web-based review sites as “top 10” performers. In addition, these four brands were readily obtained from local stores. All four e-cigarettes were “1st generation” cigarettes (i.e., generally mimicking the size and look of regular cigarettes) [11] and, with the exception of vendor D, were disposable. In addition to the e-cigarettes, their respective e-liquids (i.e., same brand, flavor, and nicotine content) were obtained.
Vendors A, B, and C indicated their claimed nicotine percentage was based on wt/wt analysis. Vendor D indicated their labeled value was based on vol/vol analysis; however, one side of the D refill solution bottle denoted 1,000 mg of nicotine, which is in keeping with a wt/wt analysis (which appears to be the industry standard) or a wt/vol analysis. Therefore, it was not entirely clear how vendor D determined their nicotine concentrations. Upon receipt, 1 mL of each e-cigarette solution was pipetted with a calibrated syringe onto a calibrated scale to determine the density of each solution. Measured densities were later used to convert wt/wt label claims to wt/vol values for direct comparison to the analytically determined wt/vol values using the following equation:
Table I: Characteristics of Electronic Cigarettes and Liquids
Vendor |
Claimed Nicotine % (wt /wt) |
Style |
Measured Density (g/mL) |
---|---|---|---|
A |
1.8 (18 mg/1,000 mg) |
Classic Tobacco |
1.1179 |
B |
1.2 (12 mg/1,000 mg) |
Classic Tobacco |
1.1843 |
C |
1.2 (12 mg/1,000 mg) |
Menthol |
1.2006 |
D |
1.8 (18 mL/1,000 mL)* |
Classic Tobacco |
1.1271 |
*One label on the solution refill bottle indicated the % nicotine was based on % vol/vol; however, the other side of the bottle denoted 1,000 mg, which is in keeping with wt/wt analysis.
Nicotine
The following system was used to analyze electronic cigarette e-liquid nicotine concentrations: an Agilent 7890A GC equipped with an Agilent FID. An Rtx-VMS column was chosen as the analytical column based on its unique ability to separate volatile compounds. The GC-FID parameters for both helium and hydrogen carrier gases are presented in Table II. The nicotine levels of the e-cigarette solutions were determined by calibrating the GC-FID with a National Institute of Standards and Technology (NIST) traceable nicotine standard (cat.# 34085). The 1,000 µg/mL nicotine standard was serially diluted with methylene chloride to generate a 7-point external calibration curve (Table III). Although not shown, a United States (U.S.) Environmental Protection Agency (EPA) Method 8260 internal standard (cat.# 30074) was found to be suitable for the current work.
All electronic cigarette solutions were diluted with methylene chloride by one hundred fold. This dilution was carried out for the following reasons: 1) Initial work with the e-cigarette solutions indicated the liquids were relatively viscous in nature. This viscosity resulted in the formation of air bubbles in the autosampler syringe. A 100:1 dilution remedied any viscosity issues. 2) The e-cigarette solutions chosen for this study appeared to have nicotine concentrations of ~15–25 mg/mL, which was outside the concentration range of the calibration curve (Table III). A 100:1 dilution resulted in nicotine levels that fell between the upper and lower limits of the calibration curve. It is important to note that methylene chloride was chosen as the diluent instead of methanol because the methanol solvent peak coeluted with the ethanol (one of the major constituents of e-cigarette solutions) peak.
Table II: Analytical system and parameters utilized for quantifying the nicotine content of electronic cigarette liquids.
Agilent 7890A GC-FID | |||
Column | Rtx-VMS, 30 m, 0.25 mm ID, 1.40 µm (cat.# 19915) | ||
Injection | Diluted (100:1) electronic cigarette liquid | ||
Inj. Vol. |
1.0 µL split (200:1) |
||
Liner |
Restek Premium Precision liner w/wool (cat.# 23305) |
||
Inj. Temp. | 250 °C | ||
Septum Purge Flow | 3 mL/min | ||
Detector |
FID @ 250 °C |
||
Carrier Gas |
He, constant flow |
H2, constant flow* |
H2, constant flow* |
Flow Rate |
2.0 mL/min |
2.50 mL/min |
2.50 mL/min |
Linear Velocity |
44.4 cm/sec |
67.2 cm/sec |
67.2 cm/sec |
Oven |
100 °C to 260 °C at 35 °C/min |
100 °C to 260 °C at 54 °C/min |
100 °C to 240 °C at 35 °C/min |
*Requires a fast ramping oven
Table III: External nicotine calibration curve for quantifying the nicotine content of electronic cigarette liquids.
1.00 mg/mL Nicotine Standard (cat.# 34085) | ||||
Level |
µL of Previous Level |
µL of Methylene Chloride |
Total Volume (µL) |
Concentration (mg/mL) |
1 |
NA |
NA |
NA |
1.00 |
2 |
100 |
100 |
200 |
0.500 |
3 |
100 |
100 |
200 |
0.250 |
4 |
100 |
100 |
200 |
0.125 |
5 |
100 |
100 |
200 |
0.063 |
6 |
100 |
100 |
200 |
0.031 |
7 |
100 |
100 |
200 |
0.016 |
Impurities
The following analytical system was used for the qualitative determination of any impurities found in the electronic cigarette solutions: an Agilent 7890B GC coupled with an Agilent 5977A MS detector. The GC-MS parameters are presented in Table IV. This analysis also utilized the Rtx-VMS column based on its proven performance for volatile compounds.
Table IV: Analytical system and parameters utilized for determination of electronic cigarette solution impurities.
Agilent 7890B/5977A GC-MS Parameters |
|
Column |
Rtx-VMS, 30 m, 0.25 mm ID, 1.40 µm (cat.# 19915) |
Injection |
Diluted (2:1) electronic cigarette liquid |
Inj. Vol. |
1.0 µL split (10:1) |
Liner |
Restek Premium 4 mm Precision liner w/wool (cat.# 23305) |
Inj. Temp. |
250 °C |
Purge Flow |
3 mL/min |
Oven |
35 °C (hold 1 min) to 250 °C at 11 °C/min (hold 4 min) |
Carrier Gas |
He, constant flow |
Flow Rate |
2.0 mL/min |
Linear Velocity |
51.15 cm/sec |
Detector |
MS |
Mode |
Scan |
Transfer Line Temp. |
250 °C |
Analyzer Type |
Single quadrupole |
Source Temp. |
230 °C |
Quad Temp. |
150 °C |
Electron Energy |
70 eV |
Tune Type |
BFB |
Ionization Mode |
EI |
Acquisition Range |
15 – 550 amu |
Rate |
5.2 scans/sec |
Vapor
Electronic cigarette vapor was analyzed for nicotine and impurities by trapping the vapor on thermal desorption tubes. Goniewicz et al. and other researchers have used smoking machines (e.g., Teague TE-2, Borgwaldt RM20S) to generate and collect e-cigarette aerosols; however, access to such an apparatus was not available for this study [7]. Therefore, in order to provide reproducible and quantitative results, a simple sampling device (Figure 1) was adapted from a 50 mL gas-tight syringe (cat.# 24761). The syringe was used to draw 40 mL of vapor in ~4 seconds from the e-cigarettes across a stainless steel thermal desorption tube packed with Tenax TA, Carbograph TD, and Carboxen 1003 (unconditioned [cat.# 26469] or conditioned [cat.# 26470]). This tube was chosen based on the optimized combination of three sorbents to screen for VOCs in the C2-3 range up to SVOCs in the C30-32 range. Although this method was manual, a ~4-second puff was utilized, as suggested based on Farsalinos et al.’s observations on e-cigarette topography [12]. In addition to the single puff sample, a 10-puff sample was also taken in order to mimic a smoking regime. This sample was taken by manually drawing ten 4-second puffs separated by 10-second intervals between puffs. The desorption tube was then transferred to the following analytical system for determining the VOCs and SVOCs directly emitted from an e-cigarette: a Markes UNITY thermal desorption system paired with an Agilent 7890B GC coupled to an Agilent 5977A MS detector. The UNITY system and GC-MS parameters are presented in Table V and Table IV, respectively.
The vapor concentrations of selected VOCs were calculated from a 5-point calibration curve generated by analyzing a series of volumes of a 10.0 ppbv primary standard (Table VI). The 10.0 ppbv primary standard was generated by injecting 180 mL of a 1.00 ppmv 75 component TO-15 + NJ mix (cat.# 34396) and 180 mL of a 1.00 ppmv ozone precursor mixture/PAMS (cat.# 34420) into an evacuated 6-liter SilcoCan air monitoring canister (cat.# 27309) and pressurizing the canister to 30 psig with 50% RH nitrogen. Ochiai et al. [13] determined 50% RH to be optimal for stability. The standard was allowed to age for 7 days.
Figure 1: Gas-tight syringe sampling apparatus for quantitatively drawing electronic cigarette vapor into a thermal desorption tube.
Table V: Markes UNITY thermal desorption system and parameters utilized for thermally extracting electronic cigarette aerosols for the qualitative and quantitative determination of VOCs and SVOCs emitted from electronic cigarettes.
Markes UNITY Parameters |
|||
---|---|---|---|
General Settings |
Trap Settings |
||
Operating Mode |
Standard two stage |
Pre-Trap Fire Purge |
1.0 min |
Standby Split |
True |
Flow |
20.0 mL/min |
Standby Flow |
5 mL/min |
Trap Low |
0 °C |
Flow Path Temperature |
210 °C |
Heating Rate |
Max |
Minimum Carrier Pressure |
5.0 psi |
Trap High |
320 °C |
GC Cycle Time |
0.0 |
Trap Hold |
5 min |
|
|
Split On |
True |
Pre-Desorption |
Split On |
20 mL/min |
|
Prepurge Time |
1.0 min |
||
Trap in Line |
False |
||
Split On |
True |
||
Flow |
20 mL/min |
||
Tube/Sample Desorption |
|||
Time 1 |
10.0 min |
||
Temperature 1 |
320 °C |
||
Trap in Line |
True |
||
Split On |
False |
Table VI: Calibration curve for calculating vapor concentrations determined on a Markes UNITY thermal desorption system.
Standard (ppbv) |
Injection Volume (mL) |
Calibration Concentration (ppbv) |
---|---|---|
10.0 |
720 |
180 |
10.0 |
360 |
90 |
10.0 |
120 |
30 |
10.0 |
40 |
10 |
10.0 |
4 |
1.00 |
Blanks
The Markes UNITY system was operated with helium carrier gas for desorbing the thermal desorption tubes and the cryogenic trap during ballistic heating for analyte focusing on the head of the analytical column. The combination of helium gas (devoid of oxygen) and elevated temperatures may have established conditions that were ideal for pyrolysis of propylene glycol and/or glycerin. The pyrolysis of propylene glycol and glycerin has been demonstrated to produce formaldehyde, acetaldehyde, and acrolein. Therefore, the following experiments were conducted to evaluate any compound contributions from the TD-GC-MS process itself: empty stainless steel tubes (i.e., no sorbents) and packed thermal desorption tubes (i.e., multi-bed sorbents) were injected with 1 µL aliquots of the electronic cigarette solutions and run through the TD-GC-MS analysis. In addition, the air drawn through the electronic cigarettes during sampling came from the laboratory. Due to the ubiquitous nature of VOCs such as formaldehyde and benzene, it was imperative to determine the background contributions of VOCs to the vapor analysis. Therefore, 40 mL samples of the laboratory air were periodically collected with thermal desorption tubes and analyzed with the same TD-GC-MS method.
Results and Discussion
Nicotine
Analyses of electronic cigarette solutions, as shown in Figure 2 (helium), Figure 3 (hydrogen, fast ramp), and Figure 4 (hydrogen, standard ramp), using the GC-FID conditions in Table II afforded the rapid (i.e., <5 minute GC run time) determination of the major chemical components. All four vendors’ e-cigarette liquids appeared to contain ethanol, propylene glycol, glycerin, and nicotine. It is important to note that all four vendors listed propylene glycol, glycerin, and nicotine; however, none of the vendors listed ethanol as an ingredient. Blank analyses indicated that ethanol was not from laboratory contamination. Methylene chloride was used as the diluent to solve viscosity and concentrations issues, hence the abundant presence of methylene chloride. As shown in Figure 5, the rapid GC-FID method produced an acceptable external calibration of nicotine from 0.016 to 1.00 mg/mL (r > 0.995).
As shown in Table VII, the vendor claimed nicotine concentrations were lower than the actual measured nicotine concentrations by 4 to 28%. Recall the wt/wt label claims were converted to wt/vol values using the measured density of each solution in order to allow direct comparison to the actual values determined analytically using the calibration curve. The observation of increased nicotine content was consistent with what Schober et al. [8] and others have observed as well.
Figure 2: Analysis of major electronic cigarette solution components via GC-FID (helium).
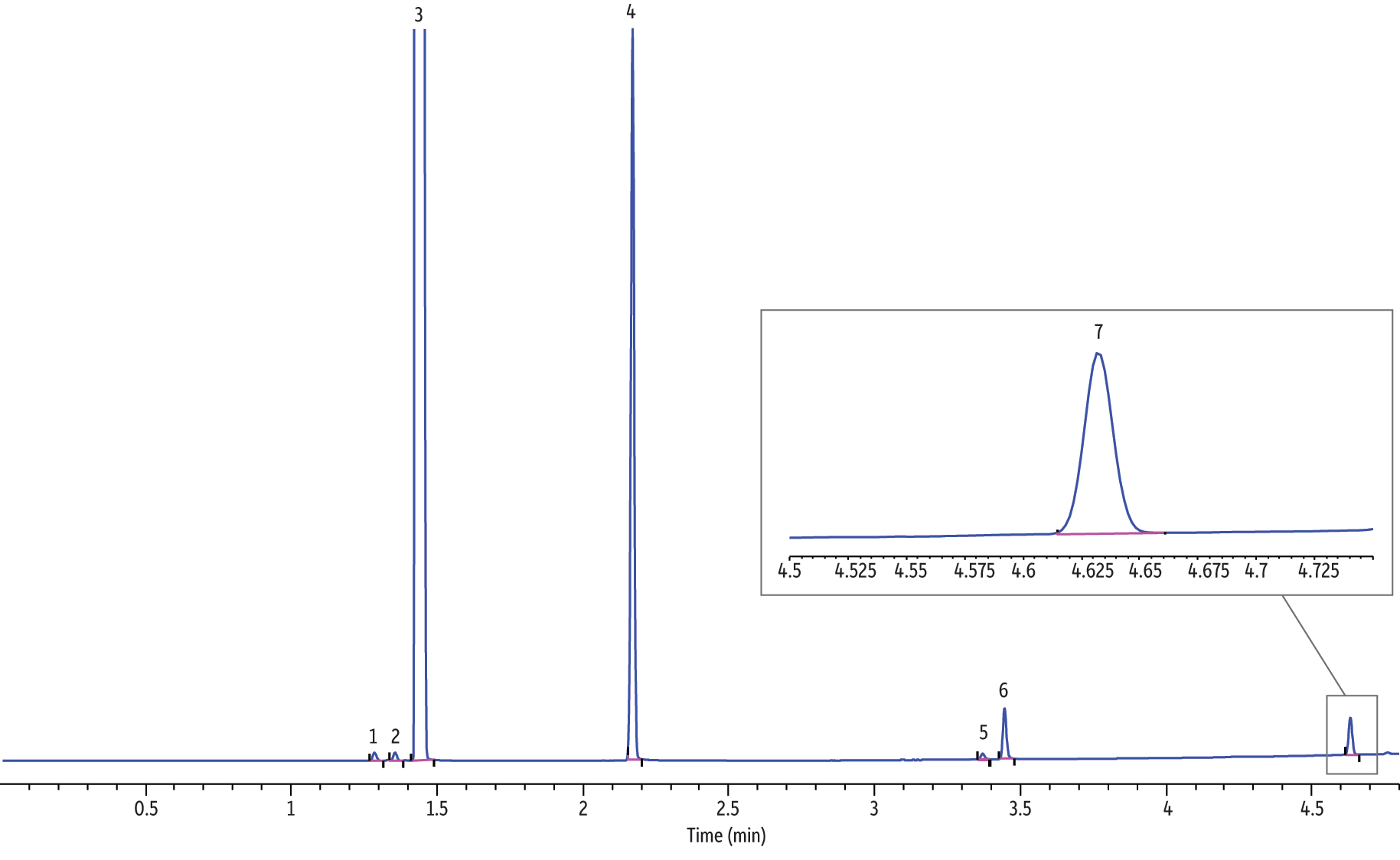
Peaks | tR (min) | |
---|---|---|
1. | Methanol | 1.285 |
2. | Ethanol | 1.355 |
3. | Methylene chloride | 1.430 |
4. | Propylene glycol | 2.174 |
5. | Unknown | 3.371 |
6. | Glycerin | 3.446 |
7. | Nicotine | 4.632 |
Column | Rtx-VMS, 30 m, 0.25 mm ID, 1.40 µm (cat.# 19915) |
---|---|
Standard/Sample | |
Diluent: | Methylene chloride |
Conc.: | Electronic cigarette liquid diluted 100:1 |
Injection | |
Inj. Vol.: | 1.0 µL split (split ratio 200:1) |
Liner: | Premium 4 mm Precision liner w/wool (cat.# 23305) |
Inj. Temp.: | 250 °C |
Oven | |
Oven Temp.: | 100 °C to 260 °C at 35 °C/min (hold 0.25 min) |
Carrier Gas | He, constant flow |
Flow Rate: | 2.0 mL/min |
Linear Velocity: | 44.4 cm/sec @ 100 °C |
Detector | FID @ 250 °C |
---|---|
Make-up Gas Flow Rate: | 50 mL/min |
Make-up Gas Type: | H2 |
Hydrogen flow: | 40 mL/min |
Air flow: | 400 mL/min |
Instrument | Agilent 7890A GC |
Figure 3: Analysis of major electronic cigarette solution components via GC-FID (hydrogen, fast ramp).
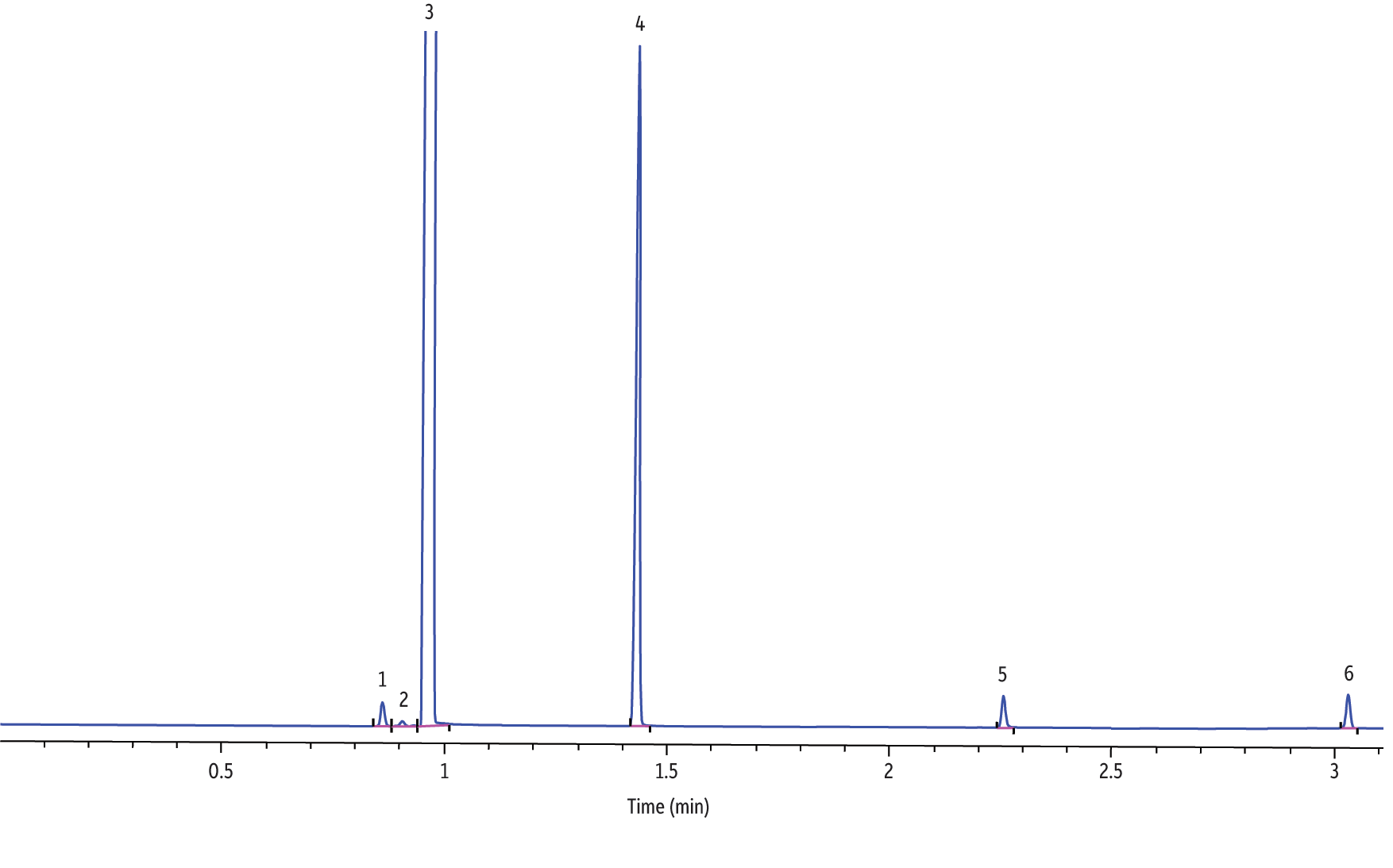
Peaks | tR (min) | |
---|---|---|
1. | Methanol | 0.861 |
2. | Ethanol | 0.905 |
3. | Methylene chloride | 0.957 |
4. | Propylene glycol | 1.433 |
5. | Glycerin | 2.256 |
6. | Nicotine | 3.030 |
Column | Rtx-VMS, 30 m, 0.25 mm ID, 1.40 µm (cat.# 19915) |
---|---|
Standard/Sample | |
Diluent: | Methylene chloride |
Conc.: | Electronic cigarette liquid diluted 100:1 |
Injection | |
Inj. Vol.: | 1.0 µL split (split ratio 200:1) |
Liner: | Premium 4 mm Precision liner w/wool (cat.# 23305) |
Inj. Temp.: | 250 °C |
Oven | |
Oven Temp.: | 100 °C to 260 °C at 54 °C/min (hold 0.15 min) |
Carrier Gas | H2, constant flow |
Flow Rate: | 2.5 mL/min |
Linear Velocity: | 67.2 cm/sec @ 100 °C |
Detector | FID @ 250 °C |
---|---|
Make-up Gas Flow Rate: | 50 mL/min |
Make-up Gas Type: | H2 |
Hydrogen flow: | 40 mL/min |
Air flow: | 400 mL/min |
Instrument | Agilent 7890A GC |
Figure 4: Analysis of major electronic cigarette solution components via GC-FID (hydrogen, standard ramp).
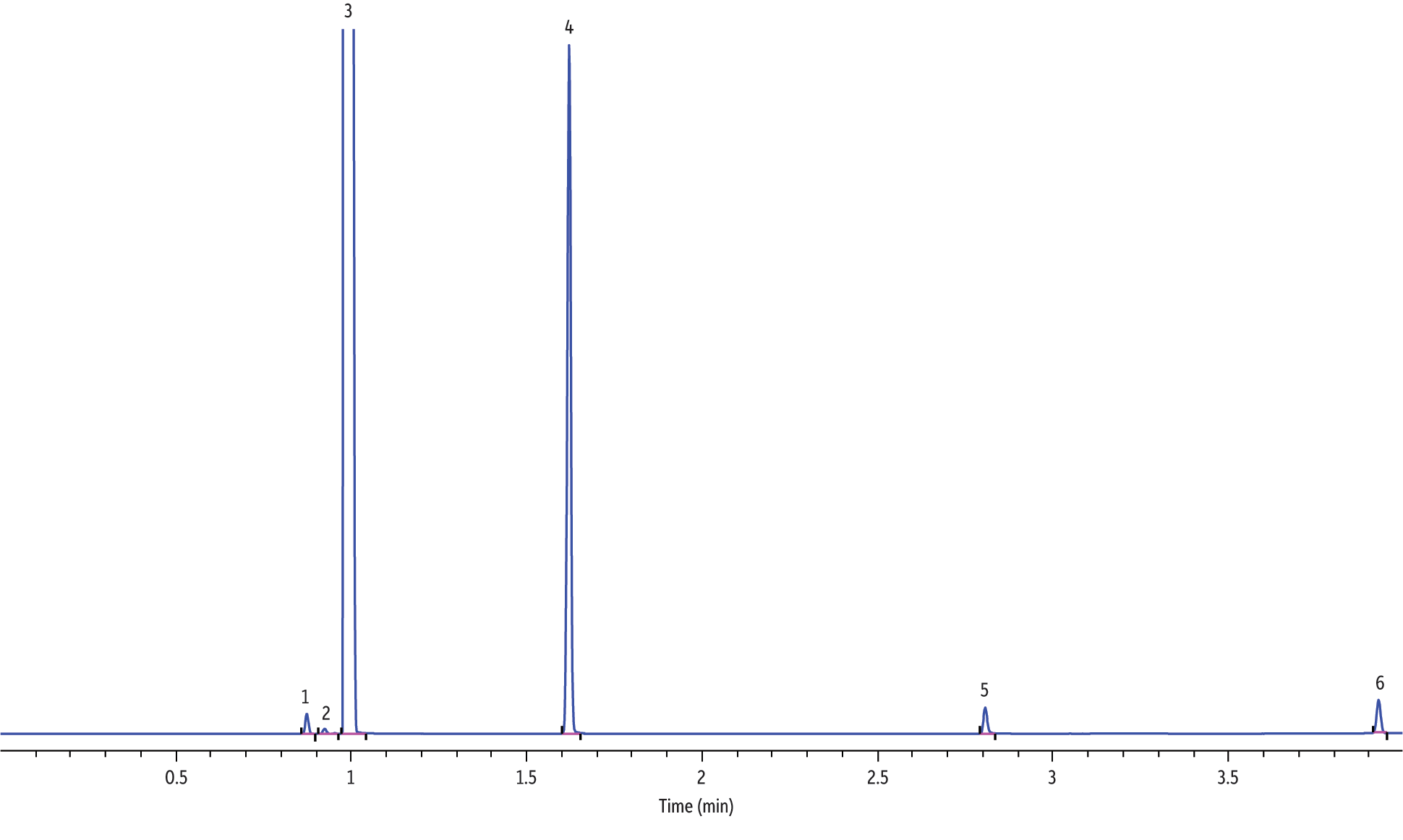
Peaks | tR (min) | |
---|---|---|
1. | Methanol | 0.875 |
2. | Ethanol | 0.926 |
3. | Methylene chloride | 0.987 |
4. | Propylene glycol | 1.619 |
5. | Glycerin | 2.805 |
6. | Nicotine | 3.927 |
Column | Rtx-VMS, 30 m, 0.25 mm ID, 1.40 µm (cat.# 19915) |
---|---|
Standard/Sample | |
Diluent: | Methylene chloride |
Conc.: | Electronic cigarette liquid diluted 100:1 |
Injection | |
Inj. Vol.: | 1.0 µL split (split ratio 200:1) |
Liner: | Premium 4 mm Precision liner w/wool (cat.# 23305) |
Inj. Temp.: | 250 °C |
Oven | |
Oven Temp.: | 100 °C to 240 °C at 35 °C/min |
Carrier Gas | H2, constant flow |
Flow Rate: | 2.5 mL/min |
Linear Velocity: | 67.2 cm/sec @ 100 °C |
Detector | FID @ 250 °C |
---|---|
Make-up Gas Flow Rate: | 50 mL/min |
Make-up Gas Type: | H2 |
Hydrogen flow: | 40 mL/min |
Air flow: | 400 mL/min |
Instrument | Agilent 7890A GC |
Figure 5: A linear response was obtained for nicotine over a concentration range of 0.06–1.00 mg/mL using the GC-FID method as demonstrated by the external calibration curve (r > 0.995).
Table VII: Vendor nicotine concentrations as claimed and as determined in the current study by direct comparison with pure nicotine standards via GC-FID.
Vendor |
Vendor Claimed Nicotine (mg/mL)^ |
Nicotine (mg/mL) Determined* |
% Difference |
---|---|---|---|
A |
20.1 |
23.4 |
17% |
B |
14.2 |
14.8 |
4% |
C |
14.4 |
17.4 |
21% |
D |
20.3 |
26.0 |
28% |
^ Calculated based on determined density.
* Average of 3 analyses.
Impurities in E-Cigarette Solutions
As shown in Figure 6, the analysis of electronic cigarette solutions revealed that they contained numerous compounds besides the vendor-listed propylene glycol, glycerin, and nicotine. For the solution shown in Figure 6 (vendor A) there were 64 unidentified and identified (some only tentatively) compounds found in the e-cigarette solution. Compounds were deemed “identified” when verified with a subsequent run of an external standard with matching retention times and mass spectral data. Compounds were deemed “tentatively identified” when the mass spectral quality was 80% or greater according to the NIST 2011 database [14]. Several pyrazines were tentatively identified, which is consistent with manufacturer-added flavorings. For example, acetylpyrazine, which was tentatively identified, is a flavorant well known for producing “nutty” flavors/aromas. In addition, several pyridines were identified, which is consistent with tobacco-derived nicotine. For example, 3-(3,4-dihydro-2H-pyrrol-5-yl)- pyridine(myosmine) was also tentatively identified and this compound is an alkaloid found in tobacco [15]. It is important to note that almost half (36) of the compounds were unidentified; future work should focus on identifying these compounds.
Figure 6: Analysis of electronic cigarette solution (e-juice) by GC-MS revealed the presence of numerous components in addition to the compounds listed on the product labels.
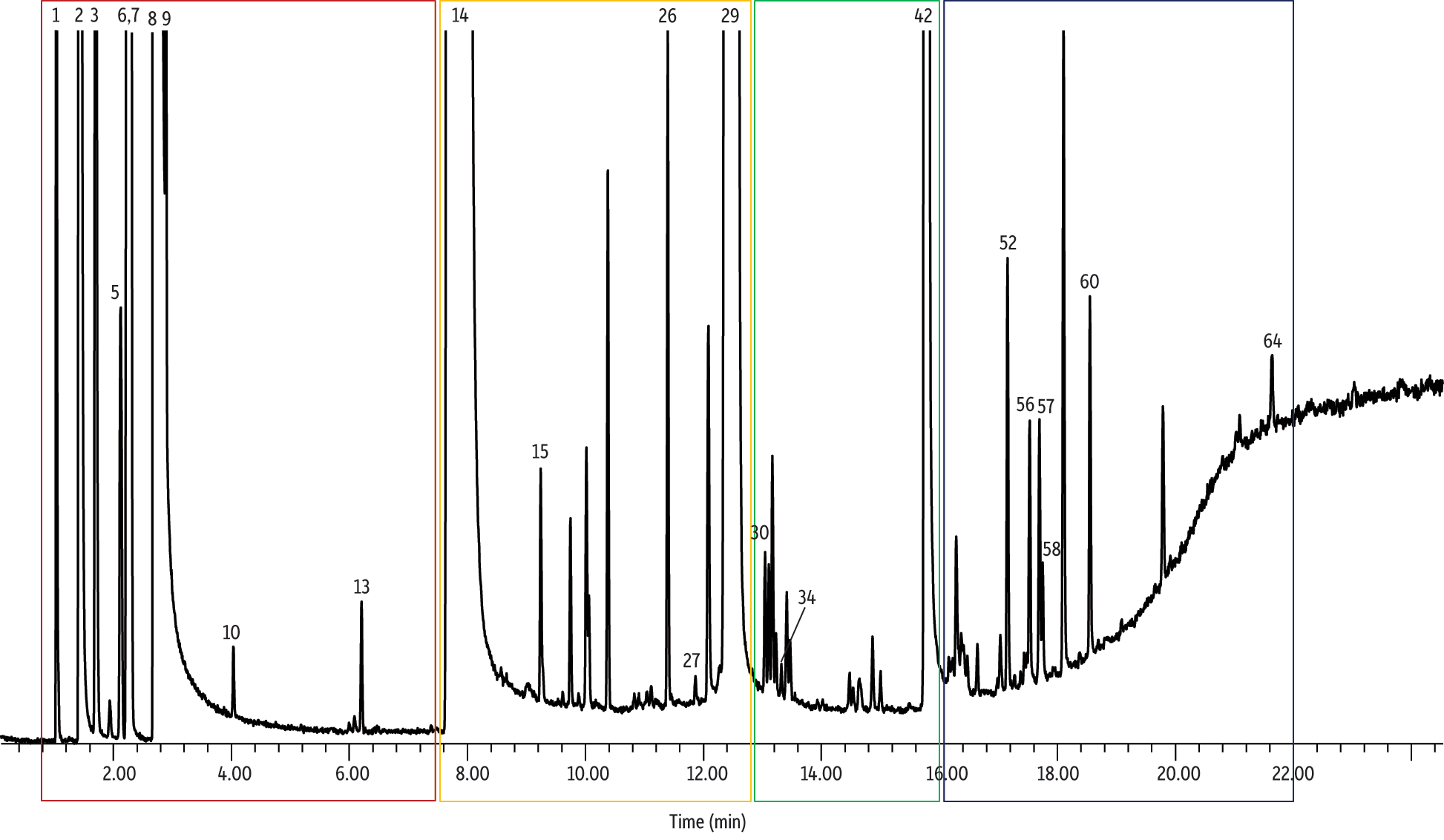
Peaks | tR (min) | Match Quality | EC Liquid | Blank | Region | |
---|---|---|---|---|---|---|
1. | Nitrogen/oxygen/carbon dioxide | 1.051 | 100 | x | x | Red |
2. | Water | 1.441 | 100 | x | x | Red |
3. | Methanol | 1.709 | 100 | x | x | Red |
4. | Unidentified | 1.934 | x | x | Red | |
5. | cis-1,2-Dimethylcyclopropane | 2.117 | 94 | x | x | Red |
6. | Ethanol | 2.239 | 100 | x | Red | |
7. | 1,1-Dichloroethene | 2.282 | 94 | x | x | Red |
8. | Methylene chloride | 2.757 | 100 | x | x | Red |
9. | 1,2-Dichloroethene | 2.891 | 94 | x | x | Red |
10. | Ethyl acetate | 4.037 | 91 | x | Red | |
11. | Unidentified | 6.000 | x | Red | ||
12. | Unidentified | 6.085 | x | Red | ||
13. | Toluene | 6.207 | 100 | x | Red | |
14. | Propylene glycol | 7.853 | 100 | x | Orange | |
15. | 2,3-Dimethylpyrazine | 9.243 | 91 | x | Orange | |
16. | Unidentified | 9.615 | x | Orange | ||
17. | Unidentified | 9.713 | x | Orange | ||
18. | Unidentified | 9.889 | x | Orange | ||
19. | Unidentified | 10.017 | x | Orange | ||
20. | Unidentified | 10.060 | x | Orange | ||
21. | Trimethylpyrazine | 10.383 | 94 | x | Orange | |
22. | Unidentified | 10.828 | x | Orange | ||
23. | Unidentified | 10.907 | x | Orange | ||
24. | Unidentified | 11.047 | x | Orange | ||
25. | Unidentified | 11.114 | x | Orange | ||
26. | Acetylpyrazine | 11.394 | 95 | x | Orange | |
27. | N-(1-Methylethyl)benzenamine | 11.864 | 80 | x | Orange | |
28. | Dipropylene glycol | 12.071 | 91 | x | Orange | |
29. | Glycerin | 12.473 | 100 | x | Orange | |
30. | Dipropylene glycol methyl ether | 13.040 | 80 | x | Green | |
31. | Unidentified | 13.107 | x | Green | ||
32. | Unidentified | 13.168 | x | Green | ||
33. | Unidentified | 13.229 | x | Green | ||
34. | 1-(3-Pyridinyl)ethanone | 13.321 | 94 | x | Green | |
35. | Unidentified | 13.412 | x | Green | ||
36. | Unidentified | 13.463 | x | Green | ||
37. | Unidentified | 14.479 | x | Green | ||
38. | Unidentified | 14.534 | x | Green | ||
39. | Unidentified | 14.643 | x | Green | ||
40. | Unidentified | 14.863 | x | Green | ||
41. | Unidentified | 15.003 | x | Green | ||
42. | Nicotine | 15.800 | 100 | x | Green | |
43. | Unidentified | 16.161 | x | Blue | ||
44. | Unidentified | 16.222 | x | Blue | ||
45. | α-Damascone | 16.289 | 95 | x | Blue | |
46. | Unidentified | 16.374 | x | Blue | ||
47. | Unidentified | 16.417 | x | Blue | ||
48. | Unidentified | 16.478 | x | Blue | ||
49. | Unidentified | 16.643 | x | Blue | ||
50. | Unidentified | 16.984 | x | Blue | ||
51. | Unidentified | 17.033 | x | Blue | ||
52. | Myosmine | 17.155 | 95 | x | Blue | |
53. | Unidentified | 17.276 | x | Blue | ||
54. | Unidentified | 17.380 | x | Blue | ||
55. | Unidentified | 17.441 | x | Blue | ||
56. | Nicotine 1-N-oxide | 17.533 | 93 | x | Blue | |
57. | Anabasine | 17.697 | 98 | x | Blue | |
58. | Nicotyrine | 17.752 | 91 | x | Blue | |
59. | Unidentified | 18.105 | x | Blue | ||
60. | 2,3-Dipyridyl | 18.550 | 97 | x | Blue | |
61. | Unidentified | 19.788 | x | Blue | ||
62. | Unidentified | 21.025 | x | Blue | ||
63. | Unidentified | 21.092 | x | Blue | ||
64. | Cotinine | 21.635 | 91 | x | Blue |
Column | Rtx-VMS, 30 m, 0.25 mm ID, 1.40 µm (cat.# 19915) |
---|---|
Standard/Sample | |
Diluent: | Methylene chloride |
Conc.: | Electronic cigarette liquid diluted 2:1 |
Injection | |
Inj. Vol.: | 1 µL split (split ratio 10:1) |
Liner: | Premium 4 mm Precision liner w/wool (cat.# 23305) |
Inj. Temp.: | 250 °C |
Oven | |
Oven Temp.: | 35 °C (hold 1 min) to 250 °C at 11 °C/min (hold 4 min) |
Carrier Gas | He, constant flow |
Flow Rate: | 2.0 mL/min |
Linear Velocity: | 51.15 cm/sec @ 35 °C |
Detector | MS | ||||||||
---|---|---|---|---|---|---|---|---|---|
Mode: | Scan | ||||||||
Scan Program: | |||||||||
| |||||||||
Transfer Line Temp.: | 250 °C | ||||||||
Analyzer Type: | Quadrupole | ||||||||
Source Type: | Extractor | ||||||||
Extractor Lens: | 6mm ID | ||||||||
Source Temp.: | 230 °C | ||||||||
Quad Temp.: | 150 °C | ||||||||
Electron Energy: | 70 eV | ||||||||
Tune Type: | BFB | ||||||||
Ionization Mode: | EI | ||||||||
Instrument | Agilent 7890B GC & 5977A MSD |
Vapor
As shown in Figure 7, the simple sampling device (Figure 1) was able to successfully draw electronic cigarette vapor into a thermal desorption tube and provide detectable levels of VOCs and SVOCs from a single 40 mL puff. As observed in the impurities study, there clearly were numerous compounds (i.e., 82 unidentified and identified [some only tentatively]) in the e-cigarette vapor beyond propylene glycol, glycerin, and nicotine. However, the analysis of the vapor revealed the presence of 18 more compounds in addition to those found in the liquid analysis. Of particular interest was the presence of formaldehyde, acetaldehyde, acrolein, and xylenes, as well as several siloxanes. The current observation of these three carbonyls (formaldehyde, acetaldehyde, and acrolein) was consistent with Goniewicz et al.’s [7] and Kosmider et al.’s [9] observations.
These observations are significant for the two following reasons: 1. All three of these carbonyls are acutely toxic; in addition, formaldehyde is a known human carcinogen [16] and acetaldehyde is a probable human carcinogen [17]. 2. These compounds were not present in the e-juice, which indicates they were generated during the vaporization process and/or from the e-cigarette materials. This is consistent with the fact that pyrolysis of glycerin results in the formation of formaldehyde, acetaldehyde, and acrolein [18]. This is also consistent with the fact that polysiloxanes are often used as plastic additives and the majority of the first generation e-cigarettes, like those evaluated in this study, are made with plastic bodies. All of the aforementioned have profound implications for how e-cigarettes should be evaluated, especially when considering that end users are ultimately exposed to the e-cigarette vapor rather than the liquid.
To expound upon this further, acrolein was not found in the electronic cigarette solutions. However, acrolein was found in the vapor from all four of the e-cigarettes evaluated in the current study. The acrolein concentrations ranged from 1.5 to 6.7 ppmv per 40 mL puff (0.003–0.015 µg/mL), which is comparable to the 0.004 µg/mL Goniewicz et al. reported [7]. To put these concentrations into perspective, these levels exceeded the National Institute of Occupational Safety and Health (NIOSH) short-term exposure limit (STEL) of 350 ppbv. Furthermore, assuming 40 mL per puff and 400 to 500 puffs per e-cigarette (values suggested by several e-cigarette manufacturers), each e-cigarette would generate ~20 to 230 µg of acrolein. From a human health perspective, the acrolein emissions observed in the current study appear to be on par with what has previously been reported for conventional tobacco cigarettes (3 to 220 µg of acrolein/cigarette) [19]. Formaldehyde and acetaldehyde standards were not available at the time of publishing this application note. However, their peak areas were on the same order of magnitude as acrolein, thereby suggesting their concentrations were comparable, which is also consistent with what Goniewicz et al. reported [7].
Currently, the U.S. Food and Drug Administration (FDA) does not have any regulatory authority over electronic cigarettes. However, the FDA does acknowledge that e-cigarettes, their associated risks, nicotine levels, and any potentially harmful chemicals inhaled are “not fully studied.” Therefore, the FDA has issued a proposed rule to extend their authority to include e-cigarettes [20]. Regardless of the status of the FDA’s authority over e-cigarettes, it is clear from the current research and the research of others that the e-cigarette landscape is not fully understood. However, it appears that e-cigarettes are not without human health risks. Most important, and as demonstrated by the current work, when designing future e-cigarette studies investigators should strongly consider the difference between analyzing electronic cigarette solutions and analyzing electronic cigarette vapor, as it very clear that their chemical profiles are different.
Figure 7: A single 40 mL puff of electronic cigarette vapor collected on a thermal desorption tube and analyzed via GC-MS.
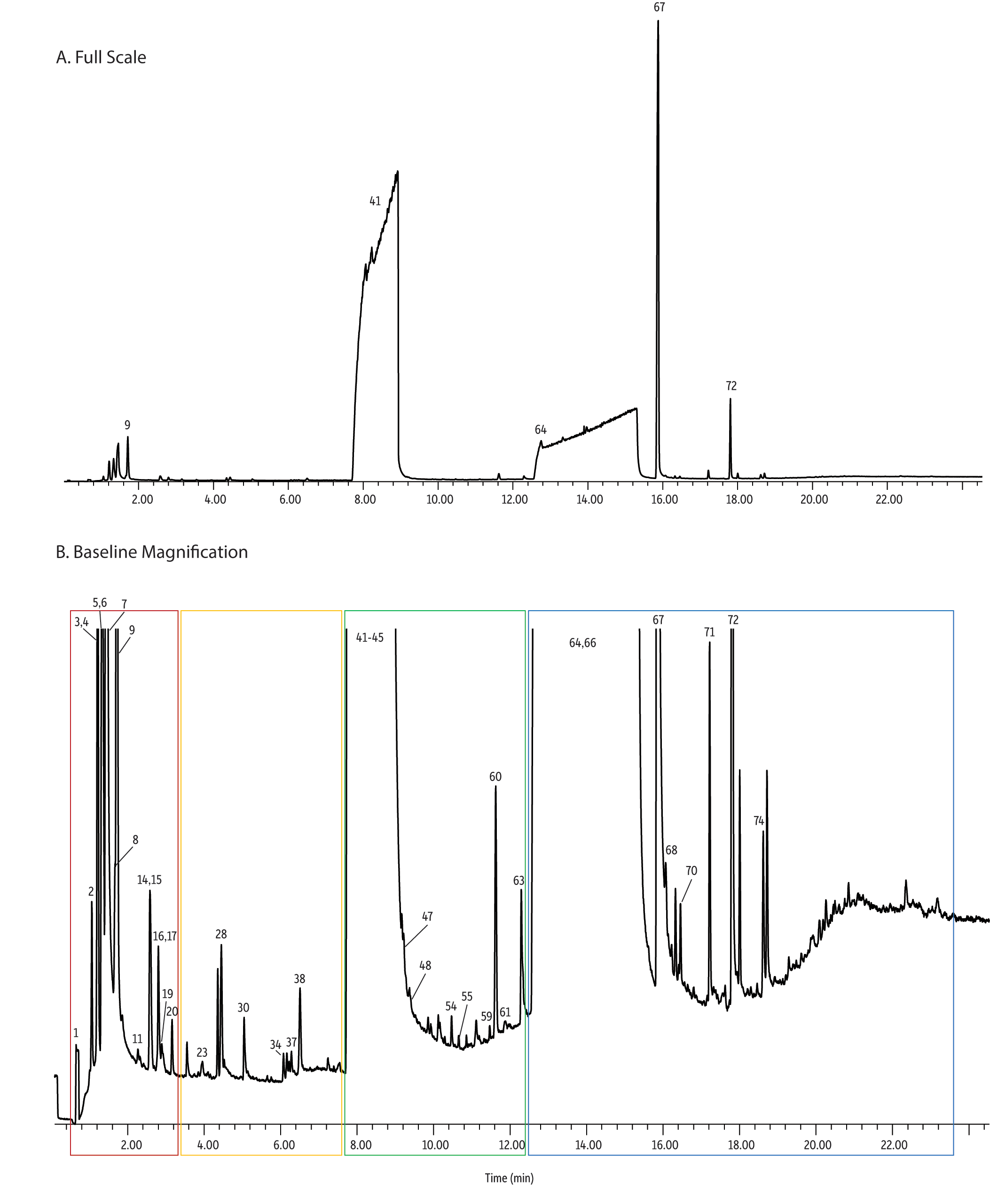
Peaks | tR (min) | Match Quality | Vapor | Blank* | Region | |
---|---|---|---|---|---|---|
1. | Nitrogen/oxygen | 0.685 | 100 | x | x | Red |
2. | Carbon dioxide | 1.063 | 100 | x | x | Red |
3. | Propene | 1.200 | 100 | x | Red | |
4. | Formaldehyde | 1.227 | 100 | x | Red | |
5. | Sulfur dioxide | 1.313 | 90 | x | Red | |
6. | Chloromethane | 1.380 | 100 | x | Red | |
7. | Water | 1.453 | 100 | x | x | Red |
8. | Acetaldehyde | 1.672 | 100 | x | Red | |
9. | Methanol | 1.715 | 100 | x | x | Red |
10. | Unidentified | 1.885 | x | Red | ||
11. | Ethanol | 2.270 | 100 | x | Red | |
12. | Unidentified | 2.331 | x | Red | ||
13. | Unidentified | 2.410 | x | Red | ||
14. | Acrolein | 2.581 | 100 | x | Red | |
15. | Propanal | 2.629 | 100 | x | Red | |
16. | Methylene chloride | 2.770 | 100 | x | x | Red |
17. | Acetone | 2.843 | 100 | x | Red | |
18. | Unidentified | 2.892 | x | Red | ||
19. | Hexane | 2.928 | 100 | x | Red | |
20. | Acetonitrile | 3.160 | 100 | x | x | Red |
21. | Unidentified | 3.544 | x | Orange | ||
22. | Unidentified | 3.842 | x | Orange | ||
23. | Trimethylsilanol | 3.928 | 100 | x | Orange | |
24. | Unidentified | 4.092 | x | Orange | ||
25. | Unidentified | 4.159 | x | Orange | ||
26. | Unidentified | 4.245 | x | Orange | ||
27. | Unidentified | 4.354 | x | Orange | ||
28. | Benzene | 4.452 | 100 | x | x | Orange |
29. | Unidentified | 4.519 | x | Orange | ||
30. | Acetic acid | 5.055 | 86 | x | Orange | |
31. | Unidentified | 5.141 | x | Orange | ||
32. | Unidentified | 5.647 | x | Orange | ||
33. | Unidentified | 5.756 | x | Orange | ||
34. | 1-Hydroxy-2-propanone | 6.073 | 80 | x | Orange | |
35. | Unidentified | 6.165 | x | Orange | ||
36. | Unidentified | 6.220 | x | Orange | ||
37. | Toluene | 6.280 | 100 | x | x | Orange |
38. | Hexamethylcyclotrisiloxane | 6.506 | 91 | x | Orange | |
39. | Unidentified | 7.231 | x | Orange | ||
40. | Unidentified | 7.530 | x | Orange | ||
41. | Propylene glycol | 7.737 | 100 | x | Green | |
42. | m-Xylene | 8.048 | 100 | x | Green | |
43. | p-Xylene | 8.048 | 100 | x | Green | |
44. | o-Xylene | 8.530 | 100 | x | Green | |
45. | Styrene | 8.597 | 100 | x | Green | |
46. | Unidentified | 9.158 | x | Green | ||
47. | Octamethylcyclotetrasiloxane | 9.218 | 91 | x | Green | |
48. | 4-Methyl-1-(1-methylethyl)cyclohexene | 9.371 | 95 | x | Green | |
49. | Unidentified | 9.639 | x | Green | ||
50. | Unidentified | 9.852 | x | Green | ||
51. | Unidentified | 9.932 | x | Green | ||
52. | Unidentified | 10.121 | x | Green | ||
53. | Unidentified | 10.219 | x | Green | ||
54. | Trimethylpyrazine | 10.468 | 80 | x | Green | |
55. | Benzaldehyde | 10.657 | 100 | x | Green | |
56. | Unidentified | 10.858 | x | Green | ||
57. | Unidentified | 11.120 | x | Green | ||
58. | Unidentified | 11.187 | x | Green | ||
59. | Acetylpyrazine | 11.541 | 93 | x | Green | |
60. | Decamethylcyclopentasiloxane | 11.620 | 91 | x | Green | |
61. | Phenol | 11.870 | 94 | x | Green | |
62. | Unidentified | 12.272 | x | Green | ||
63. | 1,1'-Oxybis-2-propanol | 12.333 | 90 | x | Green | |
64. | Glycerin | 12.748 | 100 | x | Blue | |
65. | Unidentified | 13.327 | x | Blue | ||
66. | Dodecamethylcyclohexasiloxane | 13.979 | 94 | x | Blue | |
67. | Nicotine | 15.862 | 100 | x | Blue | |
68. | Tetradecamethylhexasiloxane | 16.082 | 91 | x | Blue | |
69. | Unidentified | 16.326 | x | Blue | ||
70. | Unidentified | 16.460 | x | Blue | ||
71. | Myosmine | 17.216 | 94 | x | Blue | |
72. | Nicotyrine | 17.807 | 90 | x | Blue | |
73. | Unidentified | 18.002 | x | Blue | ||
74. | 2,3'-Dipyridyl | 18.618 | 94 | x | Blue | |
75. | Unidentified | 18.721 | x | Blue | ||
76. | Unidentified | 19.294 | x | Blue | ||
77. | Unidentified | 19.611 | x | Blue | ||
78. | Unidentified | 20.093 | x | Blue | ||
79. | Unidentified | 20.190 | x | Blue | ||
80. | Unidentified | 20.269 | x | Blue | ||
81. | Unidentified | 20.501 | x | Blue | ||
82. | Unidentified | 20.855 | x | Blue |
Column | Rtx-VMS, 30 m, 0.25 mm ID, 1.40 µm (cat.# 19915) |
---|---|
Standard/Sample | |
Conc.: | One 40 mL puff of electronic cigarette vapor drawn via a gas-tight syringe to replicate vaping |
Injection | Direct |
Oven | |
Oven Temp.: | 35 °C (hold 1 min) to 250 °C at 11 °C/min (hold 4 min) |
Carrier Gas | He, constant flow |
Flow Rate: | 2.0 mL/min @ 35 °C |
Detector | MS | ||||||||
---|---|---|---|---|---|---|---|---|---|
Mode: | Scan | ||||||||
Scan Program: | |||||||||
| |||||||||
Transfer Line Temp.: | 250 °C | ||||||||
Analyzer Type: | Quadrupole | ||||||||
Source Type: | Extractor | ||||||||
Extractor Lens: | 6mm ID | ||||||||
Source Temp.: | 230 °C | ||||||||
Quad Temp.: | 150 °C | ||||||||
Electron Energy: | 70 eV | ||||||||
Tune Type: | BFB | ||||||||
Ionization Mode: | EI | ||||||||
Instrument | Agilent 7890B GC & 5977A MSD | ||||||||
Acknowledgement | Markes |
Blanks
The 1 µL aliquots of electronic cigarette solutions injected into empty stainless steel tubes (i.e., no sorbents) and analyzed via the TD-GC-MS method resulted in the formation of formaldehyde, acetaldehyde, and acrolein. However, the concentrations of these three compounds did not increase when 1 µL aliquots of the e-cigarette solutions were injected into packed thermal desorption tubes (i.e., multi-bed sorbents) and analyzed via the TD-GC-MS method. The two aforementioned observations are consistent with the hypothesis that pyrolysis of propylene glycol and/or glycerin was taking place within the TD-GC-MS system itself and not in the thermal desorption tube media (i.e., the multi-sorbent bed). However, it was unclear as to where the pyrolysis was taking place (i.e., on the cryogenic trap during ballistic heating versus in the heated transfer lines) within the TD-GC-MS system. Regardless, the pyrolysis was responsible for 14 to 23% of the vapor concentrations of formaldehyde, acetaldehyde, and acrolein observed in the current study. The aforesaid percent contributions were approximated by comparing the carbonyl/nicotine ratios obtained from the empty stainless steel tubes and packed thermal desorption tubes to the 40 mL puff samples. In addition, the laboratory air was sometimes a source for certain VOCs; however, these levels (i.e., low ppbv) were often well below the e-cigarette levels (i.e., low to mid ppmv). Future investigators should be aware of their laboratory air concentrations and the potential pyrolysis within the TD-GC-MS system and make necessary adjustments in their reporting limits and/or background corrections. It was outside the scope of the current work; however, future work should focus on reducing pyrolysis contribution by adjusting line temperatures, heating rates, flow rates, etc.
Advantages/Limitations/Future Research
Researchers like Goniewicz et al. had access to specialized smoking machines, which enabled “realistic” smoking regimes (e.g., a 1.8 second puff with 10 second intervals between puffs). These smoking regimes may reveal more about e-cigarette vapor and/or be more accurate than the simple sampling device (Figure 1) utilized in the current study. However, the current work is significant in that multiple puffs were not needed because the present analytical techniques demonstrated detectability from a single 40 mL puff. In fact, it is important to note that a smoking regime of a 4-second puff with 10-second intervals between 10 puffs was executed manually with the simple sampling device (Figure 1). The results of this 10-puff sample are shown in Figure 8. The 10-puff sample did reveal some early eluting compounds (i.e., identified, tentatively identified, and unidentified), which were not identified in the single-puff (Figure 7). However, the propylene glycol and glycerin peaks, which were already overloaded in the single-puff sample, became so large in the 10-puff sample that most of the peaks previously identified in the single-puff sample were lost due to interference with propylene glycol and glycerin. In addition, this overloading of propylene glycol and glycerin contaminated the Markes UNITY thermal desorption system, thereby requiring a time-consuming cleaning to avoid carryover.
As previously mentioned in the discussion of the blanks results, future researchers should be aware of the potential pyrolysis conditions within the TD-GC-MS system and how that may affect their formaldehyde, acetaldehyde, and acrolein vapor concentrations. Alternative sampling/analytical approaches (e.g., DNPH-coated solid sorbents) are available for these carbonyls, which would circumvent the pyrolysis issues; however, they come at the significant disadvantage of time-consuming solvent extractions and the inability to scan for a large number of compounds (e.g., the 82 VOCs/SVOCs observed in the current study) in a single 40 mL puff. Future TD-GC-MS work on e-cigarette vapor should focus on optimizing the thermal desorption parameters in order to reduce pyrolysis contributions by adjusting line temperatures, heating rates, flow rates, etc. Overall, the current method may be well suited for the easy and rapid screening of e-cigarette vapor for a large number of VOCs and SVOCs.
Figure 8: Ten 40 mL puffs of electronic cigarette vapor collected on a thermal desorption tube and analyzed via GC-MS.
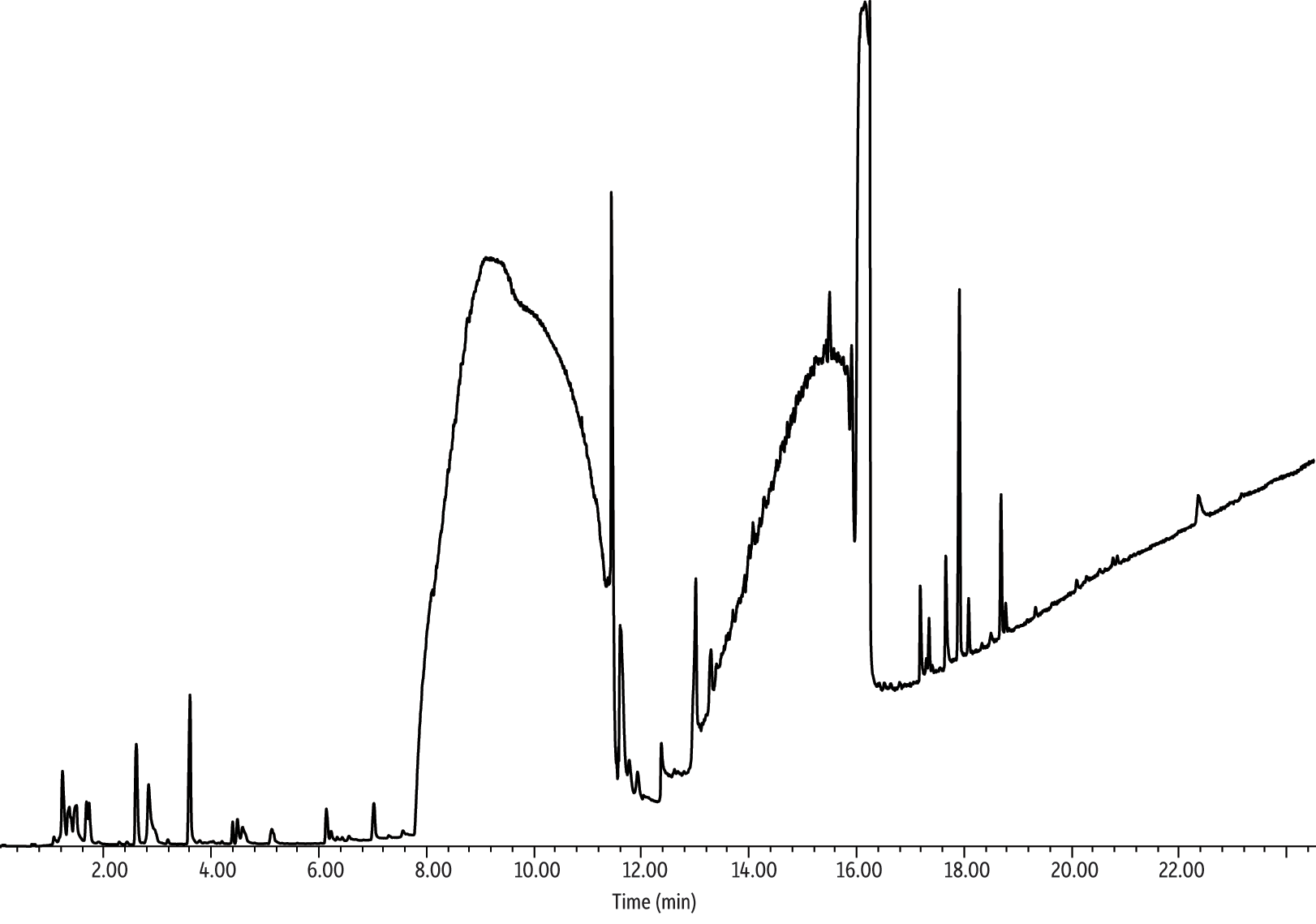
Column | Rtx-VMS, 30 m, 0.25 mm ID, 1.40 µm (cat.# 19915) |
---|---|
Standard/Sample | |
Conc.: | Ten 40 mL puffs of electronic cigarette vapor drawn via a gas-tight syringe to replicate vaping |
Injection | Direct |
Oven | |
Oven Temp.: | 35 °C (hold 1 min) to 250 °C at 11 °C/min (hold 4 min) |
Carrier Gas | He, constant flow |
Flow Rate: | 2.0 mL/min @ 35 °C |
Detector | MS | ||||||||
---|---|---|---|---|---|---|---|---|---|
Mode: | Scan | ||||||||
Scan Program: | |||||||||
| |||||||||
Transfer Line Temp.: | 250 °C | ||||||||
Analyzer Type: | Quadrupole | ||||||||
Source Type: | Extractor | ||||||||
Extractor Lens: | 6mm ID | ||||||||
Source Temp.: | 230 °C | ||||||||
Quad Temp.: | 150 °C | ||||||||
Electron Energy: | 70 eV | ||||||||
Tune Type: | BFB | ||||||||
Ionization Mode: | EI | ||||||||
Instrument | Agilent 7890B GC & 5977A MSD | ||||||||
Acknowledgement | Markes |
Conclusions
As electronic cigarettes explode in popularity, public attention is rapidly turning toward consumer safety. While research to date has focused primarily on the components of e-cigarette solutions, data presented here indicate a need for substantially more research into the chemical profile of vapor samples. To that end, this study included development of analytical methods for both solution and vapor samples. All three methods developed in the current study used an Rtx-VMS column—a proprietary phase to Restek—which was chosen to reduce required resources and afford easy comparison of results.
For e-cigarette solutions, rapid GC-FID methods using helium or hydrogen carrier gas were established for the determination of nicotine content. These methods would be suitable for fast quality control testing of electronic cigarette solutions. In addition, a straightforward GC-MS method was developed for the determination of impurities in e-cigarette solutions. Results showed that electronic cigarette solutions contained numerous compounds in addition to the compounds listed on the label by the vendor (propylene glycol, glycerin, and nicotine). In this study, e-cigarette solution profiles revealed 64 identified (some only tentatively) and unidentified compounds, far more than the three that were listed on the product label.
In order to analyze vapor samples, a simple yet novel sampling device was developed to draw electronic cigarette vapor into a thermal desorption tube, which was then thermally extracted and analyzed via a GC-MS method. This approach provided detectable levels of 82 VOCs and SVOCs from a single 40 mL puff and can be easily implemented by labs that do not have access to a smoking machine. Notably, some of compounds found are known to be detrimental to human health. These compounds were detected in the vapor, but not in the e-cigarette solution, which indicates they were produced during the vaporization process.
It is unequivocal that electronic cigarette solutions, and more important—vapor—have numerous compounds beyond the ingredients listed on the product label. As these compounds have potential implications for human health, the scientific community needs to place more emphasis on vapor testing in order to definitively identify the chemicals present and to determine how typical usage patterns relate to human health exposure limits.